Quantitative Real-Time PCR Assays of Bacterial 16S rDNA and Biomarkers for Nitrospira in Sediments of the Flint-Mammoth Cave System
science·@qiyi·
0.000 HBDQuantitative Real-Time PCR Assays of Bacterial 16S rDNA and Biomarkers for Nitrospira in Sediments of the Flint-Mammoth Cave System
A series of studies was carried out at Mammoth Cave National Park between 2001-2006 to characterize and quantify cave bacteria at several study sites. The studies were commissioned by the National Park Service to monitor microbial communities in the cave for reference in case of future contamination. Most of the data were used for internal research instead of being published in the peer review system. The study published here was the first molecular survey of Mammoth Cave bacteria. It demonstrated that clastic sandy sediments were the best substrates for study of cave bacterial communities *in situo,* as they existed in 2003. [This topic will be expanded in later posts for studies of sediments at this particular study site in Mammoth Cave called "Charon's Cascade."](https://steemit.com/science/@qiyi/quantification-and-characterization-of-a-bacterial-community-in-sediments-from-mammoth-cave) Charon's Cascade is just off the trail in the historic section of Mammoth Cave. It receives drainage from all the activity on the surface as it lies just beneath the NPS Visitor Center, hotels, campgrounds, and parking lots. In later posts, I will talk about an [E. coli outbreak at Mammoth Cave](https://www.nps.gov/maca/learn/news/ecoli-11-2006.htm) and [other research](https://steemit.com/science/@qiyi/a-day-in-the-life-of-a-cave-environmental-biotechnologist) into the 16S rDNA of Eubacterial sequences in sediments and on artificial substrates containing limestone in cave biofilms. The [Quantitative Real-Time PCR (qRT-PCR) technique was described in an earlier post](https://steemit.com/science/@qiyi/quantitative-pcr-a-brief-introduction) and in subsequent posts we will discuss the cave sediment bacteria in greater detail. Since this study showed us that cave samples are extremely variable in consistency and surface area available for biofilm growth, in later posts we will go into detail about an artificial, homogeneous sustrate we could deploy in caves anywhere. Even stable isotopes were incorporated using our artificial substrates. I breifly mentioned artificial substrates and biofilms in an [earlier post](https://steemit.com/science/@qiyi/a-day-in-the-life-of-a-cave-environmental-biotechnologist). This post describes some experimental procedures in detail, hence it will make a useful citation for later posts. **Abstract** *Quantitative Real-Time PCR was used to measure the concentration of eubacterial 16S SSU-DNA in sediments from the Flint-Mammoth Cave System (Kentucky, USA), and DNA sequence information coupled with restriction fragment biomarkers demonstrated the presence of Nitrospira in these bacterial communities. Twelve samples were collected from sites with a variety of hydrologic conditions and compared with respect to ng of bacterial DNA per g of sediment using a standard curve generated with E. coli genomic DNA. Our results demonstrate that saturated clastic stream sediments, where the processes of limestone dissolution and cavern enlargement processes are active, displayed highest bacterial DNA concentrations and the presence of a DNA sequence shared by many Nitrospira. It is concluded that Nitrospira are components of bacterial communities and can inhabit interstitial biofilms in karst aquifer clastic sediments. These chemolithotrophic organisms could contribute to cavern enlargement and to nitrogen cycling in karst aquifers.* **Introduction** The Flint-Mammoth Cave system, the longest cave on Earth, and its associated watershed are a premier example of Earth’s karst aquifers. Dissolution of soluble limestone bedrock plays a key role in the development of porosity and permeability in surface and subsurface geology of karst systems (Palmer, 1981). Globally, karst aquifers underlie some 12% of the terrestrial land surface and have been estimated to supply drinking water to 25% of the world's population. In the USA, 20% of the land surface is karst and 40% of the groundwater used for drinking comes from karst aquifers (Ford and Williams 1989; White 1988). Typically, limestone caves represent groundwater conduits that have been either abandoned by flowing water or are actively enlarging due to dissolution by flowing water (Ford and Ewers 1978, Ford and Williams 1989). Indeed, the Flint-Mammoth Cave system offers an immense underground laboratory in which to study groundwater aquifer dynamics of importance for global society, and to assess human impacts on aquifer evolution and water quality. Studies of clastic sediments and associated flowing streams at Charon’s Cascade in the historic section of Mammoth Cave demonstrated that concentrations of CO2 were tenfold higher in the interstitial fluids of the sediments than in the flowing water itself. It was suggested that elevated levels of CO2 were attributable to microbial metabolism in the sediments, and that the increased acidity contributed to acceleration of limestone dissolution measured by weight loss experiments in situ (Vaughn 1998, Vaughn et al. 1998). More detailed understanding of this phenomenon is relevant to speleogenesis (Groves 2002). Nitrifying bacteria are capable of colonizing biofilms in karst environments including epigean and subterranean streams and their sediments, sinkholes, bedrock walls, ceilings, and floors of caves and the limestone matrix itself (Fliermans and Schmidt 1977). Nitrobacter found in cave biofilms are more abundant (105 cells/gram of sediment) and tend to be of a different species than those found in the surface soils and rhizosphere according to classical microbiological techniques (Fliermans and Schmidt 1977; Hess 1900; Hill 1981). Other studies have used culturing techniques, but most cultured bacteria were likely selected by the growth conditions and some were difficult to identify (Elliott et al. 2000; Rusterholz and Mallory 1994). Modern DNA analysis techniques have revealed the existence of previously unknown bacterial species in the environment that cannot be grown under laboratory conditions in pure culture, and have revolutionized our understanding of microbial community diversity (Pace 1997). As a result, it is now known that environmental bacteria are two orders of magnitude more diverse than previously revealed by culturing techniques. Such diversity may provide strategies for microbial survival in unusual environments through interdependent communities of multiple species (Dowling et al. 1991, Hugenholz et al. 1998). DNA analysis of bacterial communities has become an important tool in understanding cave geomicrobiology (Angert et al. 1998, Barton et al. 2004, Holmes et al. 2001, Northup et al. 2000, Siering 1998). 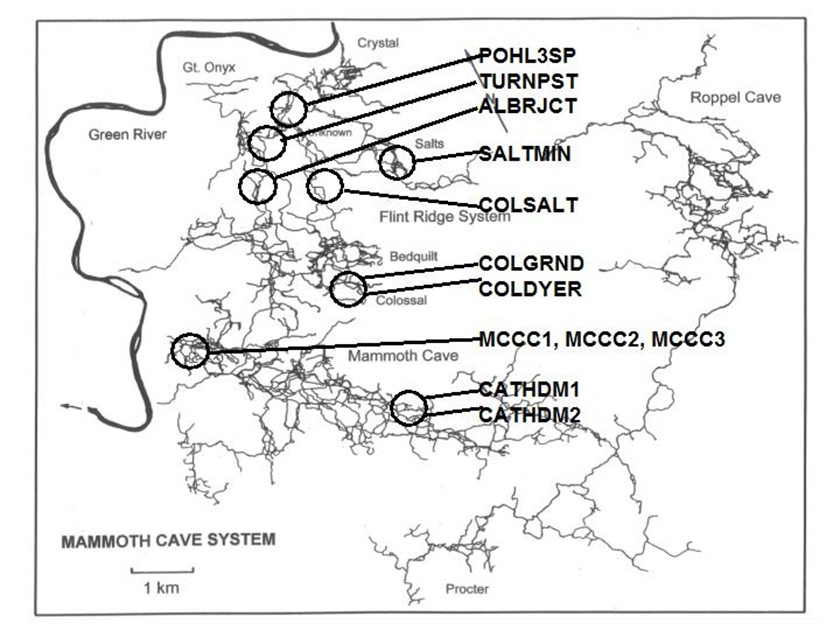 Figure 1. Map of the Flint-Mammoth Cave system showing sampling sites. Sediment samples were collected at sites outlined by circles and named as indicated. Source: modified from Palmer, 1981 by @qiyi. **Materials and Methods** *Site and sample description and collection methods* Bulk samples of 10-50 g were collected using aseptic technique, of which 0.5-1.0 g was used for DNA extraction. Samples were preserved at 4 °C until DNA was extracted from the sediment (<24 h). Twelve samples were collected from nine different sites within the Flint-Mammoth Cave system (Fig. 1). Samples of saturated clastic sediments were taken from the upper 5 cm at sites with periodic rise and fall in water level leaving deposits of fine sand. Those samples included Unknown Cave in Pohl Avenue at Three Spoons Inn (POHL3SP), Colossal Cave at Grand Avenue (COLGRND), Colossal River leading to Salts Cave along Lehrberger Avenue (COLSALT), and three samples at points within 1-5 m from the pool at Charon’s Cascade (MCCC1, MCCC2, and MCCC3). Other samples included saturated limestone paste extruding from the ceiling in Turner Avenue near Brucker Breakdown (TURNPST), two samples of accumulated sediment at the drain of a shaft complex in Cathedral Domes (CATHDM1, CATHDM2), deposits around a flood ring in Dyer’s Dome (COLDYER), dry wall deposits in the main chamber of Salts Cave containing mirabilite crystals (SALTMIN), and dry silt under a rock overhang at Albright Junction (ALBRJCT). *Extraction of DNA from sediment samples* All DNA procedures were carried out using aseptic technique. Before weighing, excess water was removed by samples were filtered to remove water by centrifugal microfiltration through a 0.2 mm membrane using a Costar #8169 spin device (Acton, MA). DNA was extracted using Ultraclean Soil DNA Kits (MO Bio Laboratories, Solana Beach, CA) by adding a known mass (0.5-1.0 g) of sediment and proceeding according to the manufacturer’s protocol. The sediment DNA was recovered in a volume of 50 μL of 10mM Tris HCl (pH 8.0) and was visualized on a 1.5% agarose gel. Volumes of 5-15 µL were used in subsequent procedures. Only one extraction was performed with each sample collected therefore standard deviations are not reported. However many manipulations were carried out in our laboratory on other sediment samples and DNA extractions (not shown) to provide confidence that the method was reliable and that the trend was consistently higher for clastic sediments (below). From 0.5-1.0 g of sediment the yield of DNA was proportioned for gel screening, one quantitative Real-Time PCR (qRT-PCR) reaction, and one fragment analysis profile. [*Quantitative Real-Time PCR*](https://steemit.com/science/@qiyi/quantitative-pcr-a-brief-introduction) Reaction mixtures consisted of 25 mL SYBR Green® Supermix (Bio-Rad Laboratories, Hercules, CA), 5 mL of 5mM eubacterial universal forward primer 27f (Lane 1991, 5’-AGAGTTTGATCMTGGCTCAG-3’), 5 mL of 5mM eubacterial universal reverse primer 1492r (Lane 1991, 5’-TACGGYTACCTTGTTACGACTT-3’), sediment DNA extracts containing up to 100 ng of template (estimated by agarose gel photoanalysis) in 5-15 µL, plus sterile nanopure water to bring final reaction volumes to 50 µL. If the DNA concentrations were too high for optimal amplification, extracts were diluted to the desired concentration range. Thermocycling and optical monitoring during qRT-PCR were performed on an iCycler Real-Time PCR machine (Bio-Rad Laboratories) with fluorescent monitoring of SYBR Green® at 490 nm during the extension step. Thermocycling conditions began with an initial denaturation for 4 min at 95° C. DNA amplification occurred during 30 cycles of 1 min at 95° C (denaturation), 1 min at 55° C (annealing), and 1 min at 72° C (extension). Reactions were brought to completion with a final extension step of 72° C for 10 min. 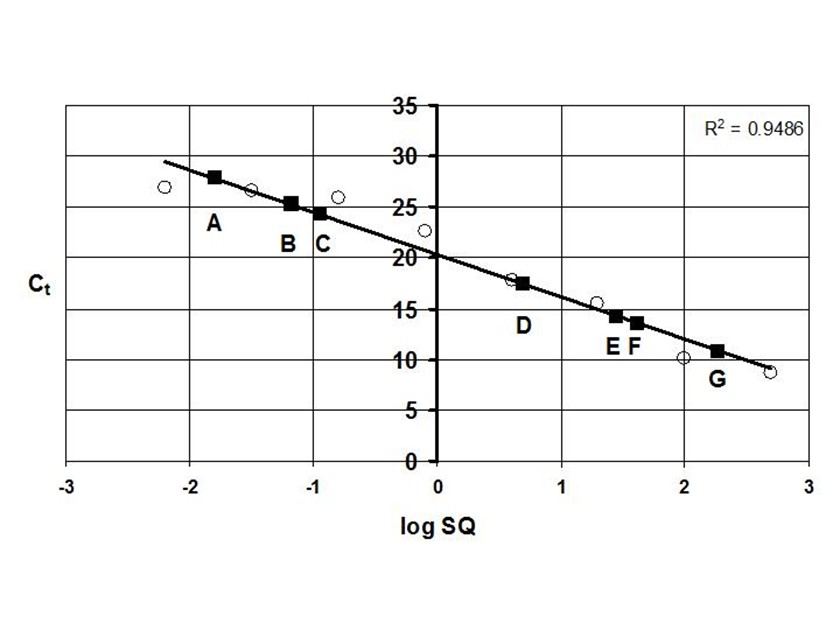 Figure 2. Graph of the threshold cycle (Ct) against the logarithm of the starting quantity of DNA (SQ) in qRT-PCR reactions. Data is shown for representative sediment DNA extracts (squares) with an E. coli standard curve (circles) and represents raw values not normalized for the mass of sediment extracted. A, SALTMIN; B, TURNPST; C, COLGRND; D, COLDYER; E, COLSALT; F, POHL3SP; G, MCCC2. Source: @qiyi. Quantitative assays measured ng of bacterial DNA contained in the 5-15 µL of extract added to the PCR reaction mixture (starting quantity, SQ) as a function of the threshold cycle (Ct) at which amplified DNA products accumulated logarithmically, correlated with an increase in SYBR-Green® fluorescence (Ponchel et al. 2003). Quantification was relative to a standard curve generated with known concentrations of E. coli genomic DNA. Unknowns plotted along with the standard curve displayed a linear relationship over a range from <0.1 ng to 100 ng of bacterial DNA added to the qRT-PCR reactions (Fig. 2). For comparison among different samples, values were normalized to represent ng of DNA per g of sediment (Fig. 3). 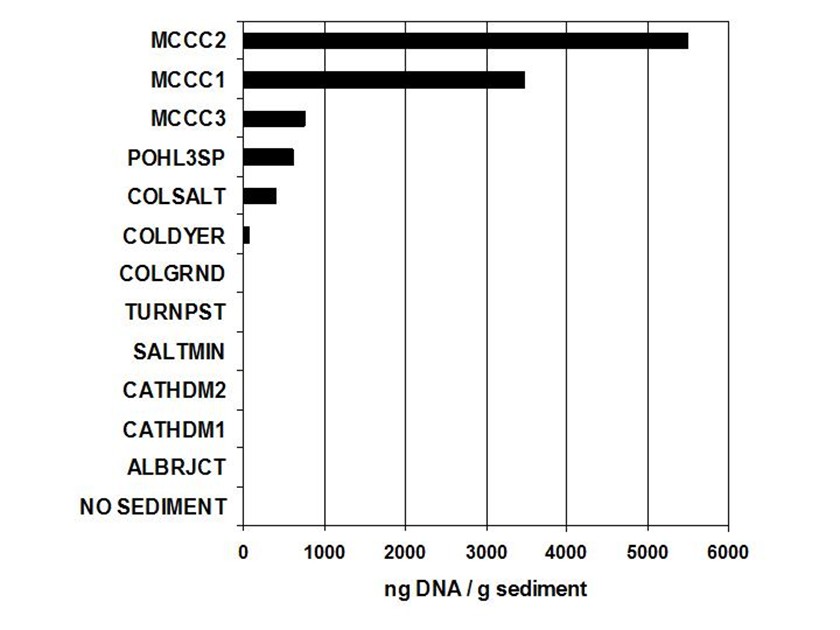 Figure 3. Bar graph showing nanograms of DNA per gram of cave sediment. SQ values from qRT-PCR results were normalized with respect to the mass of sediment from which DNA was extracted. Negative control is the product of an MOBio soil DNA kit with no sediment added. Source: @qiyi. *DNA sequencing and sequence analysis* A clone library generated from bacterial PCR products derived from MCCC1 (Sambrook, Fritsch, & Maniatis 1989) was generated for DNA sequencing of random 16S SSU-DNA genes. DNA sequencing reactions contained approximately 100 ng cloned plasmid DNA with 4 μL Big Dye Terminator mix (Applied Biosystems) and 1.6 picomoles of sequencing primer per 10 μL final reaction volume according to the manufacturer's protocol. Fluorescent capillary electrophoresis was carried out on an Applied Biosystems Prism 310 Genetic Analyzer. Overlapping fragments generated from sequencing primer sets T7:SP6, 27f:1492r, and 530f:1100r (Lane 1991) were assembled and manipulated within a database created using the Vector NTI Suite 8.0 (Informax, Bethesda, MD). Vector NTI sequence database management and analysis tools included ContigExpress for editing raw data, AlignX to perform ClustalW alignments of sequenced fragments, and BLAST searches against the GenBank nucleotide sequence database (GenBank 2003). 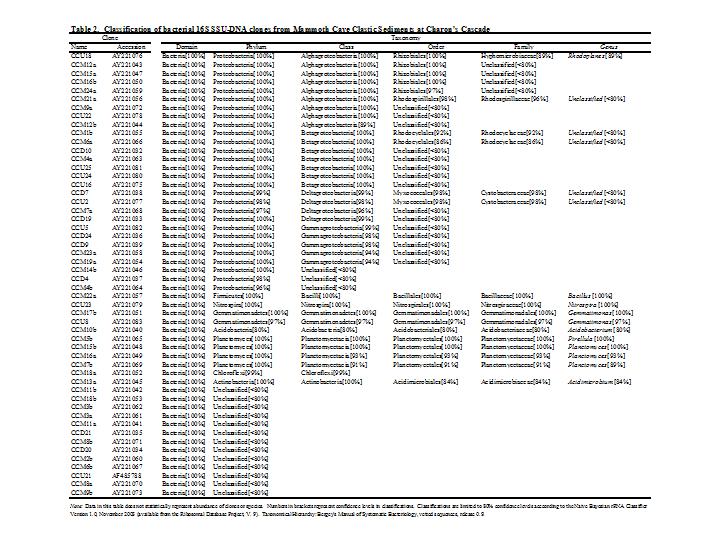 Source: @qiyi. A total of 52 sequences was compiled and examined for the closest homologous 16S SSU-DNA gene sequences from named and cultured bacteria listed on GenBank (Table above). Nucleotide sequences of the Mammoth Cave Charon's Cascade 16S SSU-DNA clones were posted on GenBank for availability to other investigators. Another set of data was posted later for both sedimets and artificial substrates. A phylogenetic analysis of the eubacterial population will be posted as well. *Fluorescent DNA fragment analysis* For detection of terminal fragments of 16S SSU-DNA, 27f primer modified at the 5’ terminus with the fluorescent label 6FAM (6FAM-27f) was used in qRT-PCR reactions. Excess primers, nucleotides, and buffers were removed with Ultraclean PCR Cleanup Kits (MO Bio Laboratories) according to the manufacturer's instructions. From the total yields of 50 μL from the PCR cleanup kits, 25 μL was cleaved with restriction enzymes HhaI (recognition sequence 5’-GCGC-3’) at 37° C or with BssHII (recognition sequence 5’-GCGCGC-3’) at 50° C. Digestion products were ethanol precipitated and resuspended in 25 μL deionized formamide (Amresco, Solon, OH) containing 1% of the internal standard fluorescent marker ROX500 (Applied Biosystems). Samples were denatured at 95° C for 4 min and quick chilled to 4° C before electrophoresis. Fragment electrophoresis, detection, and length measurement were performed an ABI 310 Genetic Analyzer running GeneScan software (Applied Biosystems). The fluorescent fragment cleaved at the recognition sequence proximal to the 6FAM reporter was detected and measured. Raw fragment peak data was refined for presentation as histograms using the Genotyper DNA profiling software package (Applied Biosystems). Fragment lengths were correlated to data obtained by the same method with database clones. Some fragments were identical to clones with known nucleotide sequences. 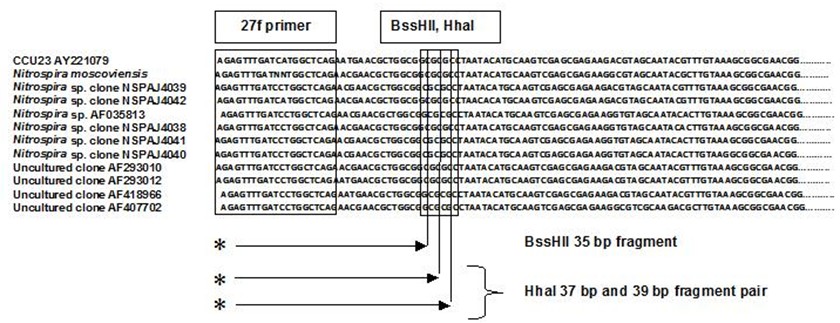 Figure 4. Alignment of approximately 100 bp of CCU23 with representative Nitrospira sequences. The 27f forward primer sequence and restriction enzyme sites are outlined. Cleavage with BssHII produces a unique fluorescent fragment of 35 bp diagnostic for CCU23 and closely related Nitrospira. HhaI produces a pair of fragments of 37 and 39 bp when cleaved at the same site. Asterisks represent 5' fluorescent label on terminal restriction fragments. Source: @qiyi. **Results and Discussion** *qRT-PCR data* Saturated clastic sediments and other moist deposits were shown to contain eubacterial 16S SSU-DNA genes in cave sediment DNA at levels from <1 to over 5000 ng of environmental DNA per g of sediment. Dry sediments tested were below qRT-PCR detection levels, suggesting bacteria were either never present or DNA was not preserved over time. Limitations to the technique derive from the fact that all bacteria do not amplify with the same efficiency with universal bacterial primers in PCR reactions, and the results may reflect some disparity for sequences contributed by species with higher or lower abundance in the actual setting. Nevertheless, this quantitative data can be used for general comparisons of nonspecific bacterial density in sediments. Highest normalized concentrations of eubacterial DNA were measured at Charon’s Cascade in the historic section of Mammoth Cave. The site receives much input from upstream tributaries and empties into River Styx, and it is subject to periodic backflooding from the Green River that results in a rich sandy deposit of clastic sediment punctuated by a waterfall approximately 10 m in height creating a permanent pool at its base (Vaughn 1998, Vaughn et al. 1998). Three samples collected around Charon’s Cascade (MCCC1, MCCC2, and MCCC3) contained 3480, 5507, and 763 ng bacterial DNA per gram of sediment, respectively. Significant DNA concentrations were measured in POHL3SP, COLSALT and COLGRND sediments with 611, 409, and 15 ng bacterial DNA per g of sediment, respectively. Cathedral Dome is subject to high drainage and limestone dissolution by flow down shaft walls (Brucker et al. 1972, Groves 2002), but sediments at CATHDM1 and CATHDM2 had concentrations of bacterial DNA below quantifiable limits. Another shaft site at COLDYER contained approximately 70 ng bacterial DNA per g of sediment which was taken from a ring above the drainage pool marking the level of a previous flood with darkened deposits, presumed to have been caused by petroleum residues. TURNPST consisted of paste on a ceiling where capillary seepage into the ceiling was sufficient to dissolve limestone, apparently extruding mineral solutes and producing a moist, gray deposit containing roughly 1 ng bacterial DNA per g of material. SALTMIN consisted of fine, dry particles with visible crystals of mirabilite containing <1 ng DNA per g of sediment. No detectable bacterial DNA was seen in ALBRJCT, despite attempts to improve detection by increasing the amount of DNA added to qRT-PCR reactions. A qRT-PCR reaction containing the product of an MOBio DNA extraction kit with no added sediment was used as a negative control (Fig. 3). *DNA sequence data* Homologous sequences extending over all or part of the length of the 16S SSU-DNA of bacteria were found by GenBank Searches. Close homologies to sequences in the library were seen with 16S SSU-DNA genes from a variety of bacterial genotypes. Sequences with the highest homologies generally were not derived from bacteria with known taxonomic classification or cultured representatives, therefore compiled data represented the closest similarities with known bacterial species identified in culture (Table above). Results have been reported at conferences (Fowler et al, 2003) and in [another post](https://steemit.com/science/@qiyi/quantification-and-characterization-of-a-bacterial-community-in-sediments-from-mammoth-cave). Sequences were examined for markers that could be used to distinguish the clones within mixtures of amplified genes by restriction enzyme cleavage alone. A clone with the required sequence features was CCU23. An alignment of the first 100 bp of CCU23 and related sequences (Fig. 5) shows similarity between CCU23 and other Nitrospira isolates and clones, many of which are not included in the alignment. Although not shown, homology extends over the full-length 16S SSU-DNA gene sequence of CCU23 (1505 bp). Inspection of the aligned sequences shows a characteristic recognition sequence for BssHII at 35 bp from the 5’ terminus and a pair of HhaI recognition sequences occupying the BssHIi recognition sequence. *Fragment analysis data* HhaI profiles revealed community diversity represented by heterogeneous fragment sizes generated by cleavage of 16S SSU-DNA sequences from many other bacteria where DNA was cleaved at a relatively common four-nucleotide sequence. However a single fragment of 35 bp was observed after BssHII digestion where a statistically less common six-nucleotide recognition sequence was cleaved (Fig. 5). 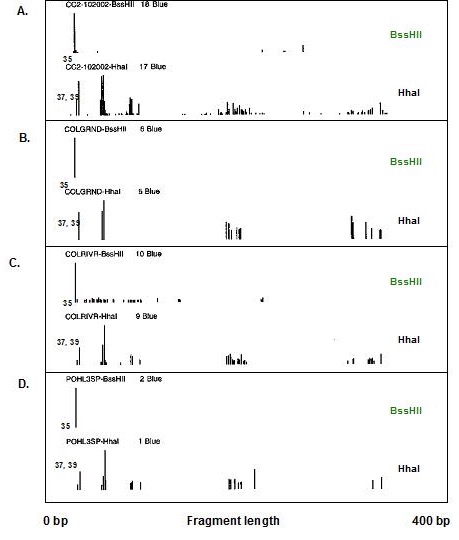 Figure 5. Histograms of fluorescent fragment profiles derived from cleavage of labeled PCR products. Cleavage with BssHII resulted in a fragment of 35 bp diagnostic for CCU23 and related Nitrospira, while cleavage with HhaI produced heterogeneous fragments representing other bacteria in the community. Fragment signals indicate presences or absences but heights of peaks are not necessarily proportional to abundances of the corresponding 16S SSU-DNA sequences. A, MCCC2; B, COLGRND; C, COLSALT; D, POHL3SP. Source: @qiyi. HhaI cleavage sites occurred with higher frequency and virtually every eubacterial 16S SSU-DNA sequence was cleaved at some position within 1500 bp from the fluorescent terminus, therefore a profile generated by HhaI cleavage displayed many fragments representing the broader bacterial community. Note also, HhaI cleaved twice within the outlined six nucleotide BssHII sequence and cleavage there resulted in a pair of DNA fragments differing in length by two bp. The combination of a 35 bp BssHII fragment with paired 37 bp and 39 bp HhaI fragments created a useful biomarker to distinguish one type of 16S SSU-DNA in the sediment bacterial community. There was no obvious correlation between HhaI fragment heterogeneity and concentration of bacterial DNA. However comparisons of fragment profiles indicated the presence or absence of some sequences at different sites. **In hindsight as written in April, 2018, this easy biomarker for a particular closely related branch of Nitrospirae has been found in many other environmental contexts. We found it in cave sediments throughout the Southeast United States when our routine screening method used this simple restriction enzyme cleavage assay. The widespread occurrence of this bacterial species with a 16S rDNA matching a particular branch of the Nitrospirae is waiting to be screened with a convenient sequence-specific test.** **Conclusions** Our data suggest that higher concentrations of bacteria were present in clastic sediments where processes of limestone dissolution and cavern enlargement are active (Brucker et al. 1972, Ford and Ewers 1978, Ford and Williams 1989, Groves et al. 1999, Groves and Meiman 1996, Thrailkill 1968, Vaughn 1998, Vaughn et al. 1998, White 1988), and that restriction fragment biomarkers diagnostic for CCU23 and other Nitrospira were detected in DNA extracted clastic sediments at speleoactive sites in the Flint-Mammoth Cave System. By contrast, highly drained sediments from shaft complexes, moist capillary seepage deposits, and sediment with crystallized mirabilite contained detectable bacterial DNA below quantifiable limits. Dry silt contained no detectable bacterial DNA. Clone CCU23 seems to be a species of Nitrospira according to 16S SSU-DNA sequencing, and its presence in various clastic sediments was demonstrated with fluorescent fragment analysis of HhaI- and BssHII-digested PCR products. Although there was no apparent predominance of Nitrospira in the clone library, other nitrifying bacteria included the ammonia oxidizer Nitrosospira (CCM1b, CCM4a, CCM6a) and the nitrite oxidizer Nitospina (CCU5). Many sequences in the library were homologous to unidentified clones taken from freshwater sediments and accretions rich in iron and manganese (Stein 2001). Nitrospira and relatives are capable of deriving energy by the oxidation of nitrite to nitrate using inorganic carbon including carbonate minerals as a carbon source (Barns and Niezwicki-Bauer 1997, Ehrich et al. 1995). Sequence-specific molecular beacon probes have been used with qRT-PCR to monitor nitrifying bacterial communities consisting of structured biofilms with Nitrosomonas, Nitrobacter, Nitrosospina, and Nitrospira responsible for the oxidation of amonia and nitrite along with decomposition of small organic molecules in municipal wastewater treatment plants and detoxifying bioreactors (Schramm et al. 2000, Burrell et al. 1998, Dionisi et al. 2002, Harms et al. 2003). Nitrifying bacteria are known to be components of corrosive biofilms (Schramm 2000). Analagous functions may be important in karst aquifer equilibrium and cave formation. It has been shown that nitrifying bacteria are associated with ferromanganese deposits in Lechugilla and Spider caves (Northup 2003). Quantitative values obtained by qRT-PCR for the mass of DNA per g of sediment are not a measure of the actual density of bacterial cells in culture, and different bacterial species differ in the size of their genomes and the number of copies of 16S SSU-DNA per cell. Also, there is bias in PCR efficiency among 16S SSU-DNA sequences. The method does provide a rapid and convenient tool for comparison of the concentrations of nonspecific bacterial DNA in sediments where culturing cells is not possible. Distinctive features of environmental 16S SSU-DNA clones can be used to develop restriction fragment biomarkers for molecular recognition of specific bacteria in cave sediments without the need to isolate and culture the organisms. **Acknowledgments** This work was supported by a cooperative agreement between Mammoth Cave National Park (MACA) and Western Kentucky University (WKU). All work was carried out in the facilities of the Biotechnology Center, which is part of the Applied Research and Technology Program (ARTP) at WKU. **References** [Angert, E.R., Northup, D.E., Reyesenbach, A.-L., Peek, A.S., Goebel, B.M., & Pace, N.R., 1998, Molecular phylogenetic analysis of a bacterial community in Sulphur River, Parker Cave, KY: American Mineralogist, v. 83, p. 1583-1592.](http://www.minsocam.org/msa/AmMin/TOC/Articles_Free/1998/Angert_p1583-1592_98.pdf) Barnes, S.M. & Nierzwicki-Bauer, S.A. 1997, Microbial diversity in ocean, surface, and subsurface environments: in J.F. Banfield and K.H. Nealson, (eds.), Geomicrobiology: Interactions between microbes and minerals, Reviews in Mineralogy: American Minerological Society, Washington, DC, v. 35 p. 35-71. [Barton, H.A., Taylor, M.R., & Pace N.R., 2003 phylogenetic analysis of a bacterial community in an oligotrophic cave environment: Geomicrobiology Journal, v. 21, p. 11-20.](https://www.tandfonline.com/doi/abs/10.1080/01490450490253428) Brucker R.W., Hess, J.W., &. White, W.B., 1972, Role of vertical shafts in the movement of ground water in carbonate aquifers: National. Speleological Society Bulletin, v. 10, no. 6, 9 pp. Burrell P.C., Keller J., & Blackall L.L., 1998, Microbiology of a nitrite-oxidizing bioreactor: Applied and Environmental Microbiology, v. 64, no. 5, p. 1878-1883. Dionisi, H.M., Layton A.C., Harms, G., Gregory, I.R., Robinson, K.G., and Sayler, G.S., 2002, Quantification of Nitrosomonas oligotropha-like ammonia-oxidizing bacteria and Nitrospira species from full-scale wastewater treatment plants by competitive PCR, Applied and Environmental Microbiology, v. 68, no. 1, p. 245-253. Dowling, N.J.E., Mittelman, M.W., & White, D.C., 1991, The role of consortia in microbially influenced corrosion: in J. G. Zeikus, (ed.), Mixed Culture in Biotechnology, McGraw Hill, NewYork, NY, p. 341-372. Elliott, L., Wright, S., Coakley, T., & Groves, C., 2000, Microbial ecology of conduit stream interstital fluids of the southcentral Kentucky karst aquifer: Impacts on aquifer development: Proceedings of Mammoth Cave National Park's Eighth Science Conference, p. 57-60. [Ehrich, S., Behrens, D., Lebedeva, E., Ludwig, W., & Bock, E., 1995, A new obliagtely chemolithoautotrophic, nitrite-oxidizing bacterium, Nitrospira moscovienses sp. no. and its phylogenetic relationship: Arhives of Microbiology, v. 164, p. 16-23.](https://www.ncbi.nlm.nih.gov/m/pubmed/7646315/) Fliermans, C.B., & Schmidt, E.L., 1977, Nitrobacter in Mammoth Cave: International Journal of Speleology, v. 9, p. 1-19. Ford, D.C. & Ewers, R.O., 1978, The development of limestone cave systems in the dimensions of length and depth: Canadian Journal of Earth Science, v. 15, p. 1783-1798. Ford, D.C. & Williams, P., 1989, Karst geomorphology and hydrology, Unwin Hyman Ltd., Winchester, MA, 601 pp. [Fowler, R., Roberson, E., and Sahi, S. (2003). Quantitative Real-Time Assays of Bacterial DNA in Sediments of the Flint-Mammoth Cave System with evidence for Nitrospira spp. at sites Undergoing Limestone Dissolution and Karst Aquifer Evolution. Proceedings of the 16th Annual National Cave and Karst Management Symposium, Gainesville, FL, Oct. 13-17.](http://www.nckms.org/2003/pdf/FOWLER.pdf) GenBank, 9 Jul 2003, NCBI BLAST: National Center for Biotechnology Information, http://www.ncbi.nlm.nih.gov/genbank Groves, C. 2002. Personal communications. Groves, C. & Meiman, J., 2001, Inorganic carbon flux and aquifer evolution in the South Central KY karst: U.S. Geological Survey Karst Interest Group Proceedings, St. Petersburg, FL, Feb. 13-26, 2001, Water-Resources Investigations Report, 01-4011, p. 99-105. Groves, C. & Meiman, J., 1996, What are we learning at the Hawkins River Site? Proceedings of the Fifth Mammoth Cave Science Conference, p. 131-136. Groves, C., Meiman, J. & Howard, A.D. 1999, Bridging the gap between real and mathematically simulated karst aquifers: Invited paper for the Proceedings of the Karst Waters Institute International Symposium on Karst Modeling, Charlottesville, VA, p. 197-202. [Harms, G., Layton, A.C., Gregory, I.R., Garrett, V.M., Hawkins, S.A., Robinson, K.G., & Sayler, G.S., 2003, Real-Time PCR quantification of nitrifying bacteria in a municipal wastewater treatment plant: Environmental Science and Technology, v. 37, p. 343-351.](https://www.ncbi.nlm.nih.gov/m/pubmed/12564907/) Hess, W.H. 1900, The origin of nitrates in cavern earths: Journal of Geology, v. 8, p 129-134. Hill, C.A., 1981, Origin of cave saltpeter: National Speleological Society Bulletin, v. 43, p. 110-126. Holmes, A.J., Tujula, N.A., Holley, M., Contos, A., James, J.M., Rogers, P., & Gillings, M., 2001, Phylogenetic structure of unusual aquatic formations in Nullarbor caves, Australia: Environmental Microbiology, v. 3, no. 4, p. 256-264. [Hugenholtz, P., Pitulle, C., Hershberger, K.L., & Pace, N.R., 1998, Novel division level diversity in a Yellowstone hot spring: Journal of Bacteriology, v. 180, no. 2, p. 366-376.](https://www.ncbi.nlm.nih.gov/pmc/articles/PMC106892/) Lane, D.J., 1991, 16S/23S rRNA Sequencing: in E. Stackenbrandt and M. Goodfellow (eds), Nucleic acid techniques in bacterial systematics, John Wiley and Sons, Inc. New York, NY, p. 115-148. [Northup, D.E., Barns, S.M., Yu, L.E., Spilde M.N., Schelble, R.T., Dano K.E., Crossey L.J., Connolly, C.A., Boston P.J., Natvig, D.O., & Dahm, C.N., 2003, Diverse microbial communities inhabiting ferromanganese deposits in Lechuguilla and Spider Caves, Environmental Microbiology v. 5 no. 11 p. 1071-1086.](https://www.ncbi.nlm.nih.gov/m/pubmed/14641587/) Northup, D.E., Dahm, C.N., Melim, L.A., Spilde, M.N., Cossey, L.J., Lavoie, K.H., Mallory, L.M., Boston, P.J., Cunninhamm, K.I., & Barns, S.M.,2000, Evidence for geomicrobial interactions in Guadalupe Caves: Journal of Cave and Karst Studies, v. 62, no. 2, p. 80-90. Pace, N.R., 1997, A molecular view of microbial diversity in the biosphere: Science, v. 276, p. 734-740. Palmer, A.N., 1981, A geological guide to Mammoth Cave National Park: Teaneck, N.J., Zephyrus Press, 210 pp. Ponchel. F., Toomes, C., Bransfield, K., Leong, F.T., Douglas, S.H., Field, S.L., Bell, S.M., Combaret, V., Puisieux, A., Mighell, A.J., Robinson, P.A., Ingelehearn, C.F., Isaacs, J.D., & Markham, A.F., 2003, Real-Time PCR based on SYBR-Green I Fluorscence: An alternative to the TaqMan assay for a relative quantification of gene rearrangements, gene amplifications, and micro gene deletions, BMC Biotechnology, v. 3, no. 18. Rusterholtz, K.J. & Mallory, L.M., 1994 Density, activity, and diversity of bacteria indigenous to a karstic aquifer: Microbial Ecology, v. 28, p. 79-99. Sambrook, J., Fritsch, E.F., & Maniatis, T., 1989, Molecular cloning, a laboratory manual (2nd edition): Cold Spring Harbor Laboratory Press, v. 1, p. 1.53-1.86. Schramm, A., De Beer, D., Gieseke, A., & Amann, R., 2000, Microenvironments and distribution of nitrifying bacteria in a membrane bound biofilm: Environmental Microbiology v. 2, no. 6, p. 680-686. Siering, P.L., 1998, The double helix meets the crystal lattice: The powers and pitfalls of nucleic acid approaches to mineralogical investigations: American Mineralogist v. 83, no. 11-12, Part 2, p. 1593-1607. Stein, L.Y., La Duc,M.T., Grundl,T.J. & Nealson,K.H., 2001, Bacterial and archaeal populations associated with freshwater ferromanganous micronodules and sediments, Environmental Microbiology, v. 3, no. 1, p. 10-18. Thrailkill, J., 1968, Chemical and hydrologic factors in the excavation of limestone caves, Geological Society of America Bulletin. v. 79, p. 19-46. Vaughan, K., 1998, A quantitative analysis of interstitial fluid chemistry and limestone dissolution rates within the clastic sediment of a karst aquifer conduit, Mammoth Cave, KY, M.S. Thesis, Western KY University, 128 pp. Vaughan, K., Groves, C., & Meiman, J. 1998, Carbonate chemistry and interstitial fluids within cave stream sediments: in Proceedings of the International Geological Correlation Program, Project 379: “Karst processes and the global carbon cycle,” Bowling Green, KY. White, W.B., 1988, Geomorphology and hydrology of karst terrains: Oxford University Press, New York , NY, 464 pp.
👍 filipino, magpielover, moby-dick, qiyi, sensation, vinamra, micro24, davidrhodes124, aboutcoolscience, elenakravchenko, romanis, martakent, iramanetz, mobbs, steemstem, anarchyhasnogods, lafona-miner, justtryme90, hadji, lemouth, flurgx, joseg, felixrodriguez, simplifylife, mayowadavid, erodedthoughts, enzor, robotics101, tristan-muller, sco, adetola, jlmol7, mittymartz, rionpistorius, kingabesh, ajpacheco1610, anyes2013, simplicitytech, effofex, count-antonio, de-stem, temitayo-pelumi, beautyinscience, rbm, ari16, fredrikaa, abigail-dantes, borislavzlatanov, dysfunctional, thevenusproject, the-devil, mountain.phil28, monie, deutsch-boost, croctopus, jaycem, doctor-cog-diss, funster, biomimi, dna-replication, curie, foundation, locikll, gambit.coin, himal, leczy, xanderslee, markangeltrueman, tantawi, aboutyourbiz, howtostartablog, zacherybinx, phogyan, birgitt, spectrums, motivatorjoshua, musicayfarandula, speaklife, victoryudofia, anwenbaumeister, ihsan19, jacalf, karyah1001, esaia.mystic, crescendoofpeace, geekis, randomwanderings, loydjayme25, kimp0gi, chimtivers96, star-vc, lamouthe, hendrikdegrote, makrotheblack, skycae, kekegist, strings, dyancuex, ertwro, wandersells, karolisp, pacokam8, juanjdiaz89, infinitelearning, raoul.poenar, fibrefox, dashfit, muliadi, vadimlasca, smafey, rachelsmantra, laritheghost, jamhuery, lrsm13, nitesh9, bobdos, echavez82, debbietiyan, ylich, phaazer1, chillingotter, ikeror, sethroot, mahdiyari, churchboy, runningman, paulthebeloved, rasamuel, cerventus, aaronteng, qberry, kushed, kerriknox, onethousandwords, jpmkikoy, caitycat, etaletai, jgpro, meetmysuperego, sireh, mathowl, victorcovrig, qanon1111, soundworks, boynashruddin, saunter, clweeks, steempeninsula, loudetteiam, justinmullet, ejhaasteem, misterakpan, opheliapoe, digitalpnut, mountainjewel, saunter-pl, faithcalls, tasjun, carolynseymour, ghostgtr, zeeshan003, steem-hikers, kendallron, steem.curator, ewuoso, fischkopp, pangoli, physics.benjamin, sakura1012, serylt, nedspeaks, gra, lenin-mccarthy, positiveninja, chloroform, rockeynayak, tormiwah, rjbauer85, ugonma, wisewoof, dexterdev, akeelsingh, suravsingh, kryzsec, sci-guy, amavi, dber, imamalkimas, gentleshaid, blessing97, kenadis, carloserp-2000, wildmanhowling, the-naked-geek, jdc, wdoutjah, mindscapephotos, damzxyno, thinknzombie, massivevibration, happychild, benleemusic, helgapn, onartbali, ntowl, torico, coloringiship, jaeydallah, bennettitalia, aamin, steepup, apteacher, vanessahampton, aehiguese, ninyea, delph-in-holland, theunlimited, alinabarbu, thatterrioguy, peaceandwar, cordeta, fidelpoet, aljoursantillan, scortan, tking77798, alexander.alexis, steemittopfan, aloader, ilikesteemit,